From the Last Skirmishes around the Neuron Theory to the Functional Anatomy of Neuron Networks
John Szentágothai
Reticularism or the Neuron Concept
When I was "awakening" on the scene of neurobiology in the early thirties, the neuron concept was once again under heavy barrage from the "reticularists", then led by Jan Boeke at Utrecht and by Philipp Stöhr, Jr., at Bonn. My first impressions about this nineteenth-century type of scientific Streitschrift (debate) derived from the hopeless struggle of my revered teacher in anatomy, Michael von Lenhossék, to refute the claims of Boeke (1926) about the existence of a "periterminal network" in the motor endplate, which would allegedly prove the continuity between neural and muscle substance. Von Lenhossék had too critical a mind not to realize that it was impossible then to beat Boeke with his own weapons, the sole use of neurofibrillar impregnation methods: so he refrained from publishing anything about the results of his last researches. However, I became "imprinted" with a fundamental distrust of the "histological picture" as a source of information about things that were not obvious in routine preparations and not visible with the mediocre resolving power of available optic systems.
A beginner like myself was, of course, not supposed to meddle in such high-level affairs, so I was commissioned by von Lenhossék to study the connections of the geniculate ganglion. I immediately became fascinated with the possibilities offered by experimental (Wallerian) degeneration and by cell and fiber counts (Schimert, 1936b).1 Very soon, however, my interests shifted to another field: the structure of the vegetative nerve terminals, which at the time was the main battlefield of the "reticularists." The apparently reticular structures labeled Grund-plexus (ground plexus) by Boeke (1933a,b) and Terminalreticulum by Stöhr (1935) were considered as clear evidence that neurofibrillar structures could invade other cells, mainly Schwann cells, and (according to Stöhr) also other tissues. The histological pictures furnished by various modifications of the Bielschowsky method were indeed deceptive, and I might have succumbed to the beauty of the seemingly reticular structures and the bombastic rhetoric of the chief reticularists had it not been for the advice of my immediate laboratory chief, P. von Mihálik, a somewhat strange personality but a man of exceptional clarity of mind and an unusual knowledge and sound criticism of the cytological and histological literature.
Having had some experience with experimental secondary degeneration, I soon concluded that of the two principal options - one, that the silver-stained filamentous elements were neurofibrils, as claimed by the "reticularistsr" or, two, that they were axons, as assumed by the "neuronists"- option one was not disproved by degeneration occurring after the removal of ganglia supplying the terminal plexus (secondary degeneration in vegetative terminals had been shown in 1934 by Lawrentjew and several of his coworkers). However, option two would and could be proven exclusively correct if it could be shown that the filamentous elements underwent partial degeneration in the same Schwann-cell process after partial transection of the nerves feeding into the network.
The experiments showed very clearly that some impregnated filaments underwent degeneration, while others in the same Schwann cell did not (Figure 6.1A; see Schimert, 1936a,1937,1938a). The conclusion was that these elements were nerve fibers (axons) from various sources, with up to ten or more axons embedded in the same Schwann-cell process.
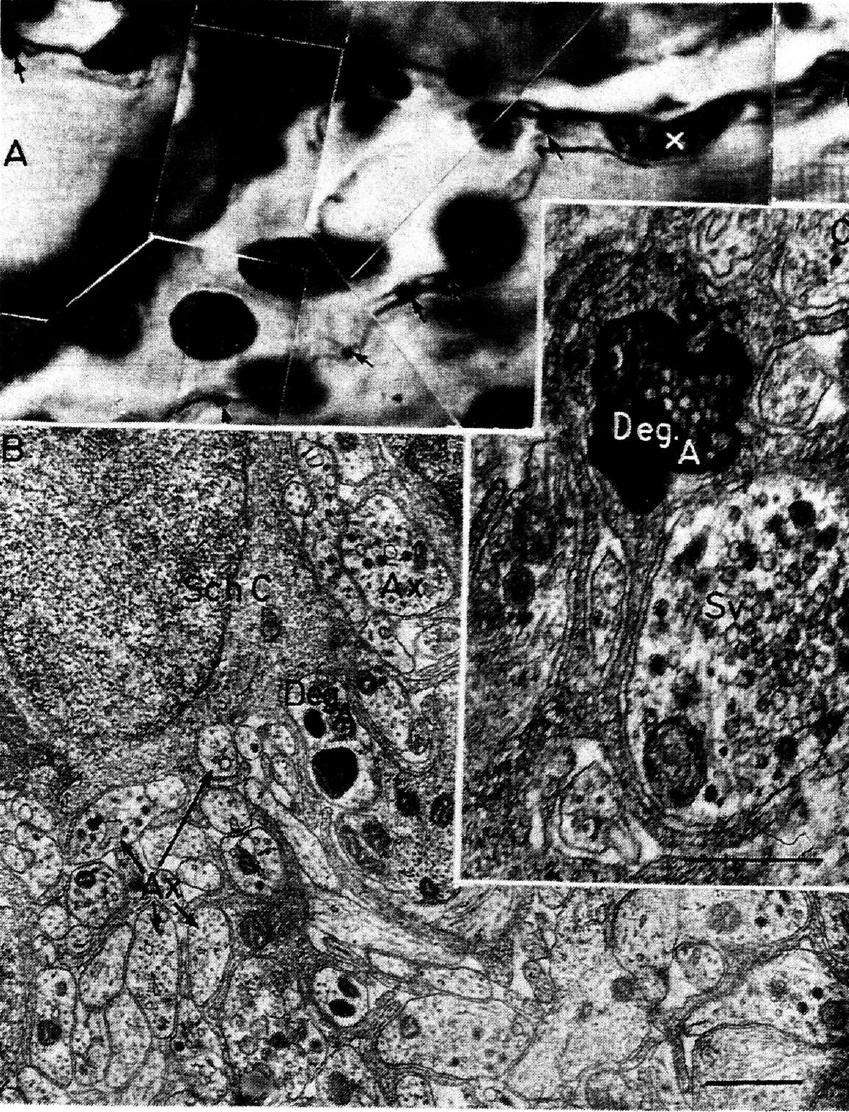
Fig. 6.1. A comparison between the degeneration evidence, available on the light-microscopic
level in the mid-thirties, and as seen today under the electron microscope. (A) A partly degenerated
"ground plexus" in the myocardium of the cat three days after removal of one stellate ganglion. The degeneration fragments are clearly visible as black dots (arrows) together with normal
axons in the same Schwann-cell process (x marks the Schwann-cell nucleus). From Schimert
(1937). (B) The honeycomb structure of a Schwann cell (Sch. C.) with numerous small axons (Ax)
embedded. One axon is in the early so-called cytolysomal stage of degeneration. (C)The same area
under somewhat higher power, where besides normal axons (one with varicose thickening
showing accumulation of synaptic vesicles = Sv), a fiber fragment is visible in later stage of degeneration (Deg. A). From an intestinal terminal plexus and thematerial of Ungváry and Léránth. The evidence, hence, was as good almost forty years ago as it is today; however, practically
nobody cared for it.
Needless to say, my humble efforts did not at all impress the "reticularist establishment" of the time, beyond some remarks about the "childish attempts of some nincompoop beginner" (see Stöhr, 1939: pp. 603-604). It is fair, though, to mention that the situation was this bad only in continental European histology in American and Soviet neurohistology, reticularist views exercised less influence. Nevertheless, I was the only man who stood up in defence of the neuron theory when the Anatomische Gesellschaft, at the 1937 Congress at Königsberg, sought to "bury the neuron theory" as announced by Boeke in his characteristic flowery speech.
It is not exactly gratifying to find that now, when this issue has been clarified beyond any doubt through electron microscopy (Figure 6.1B and C), hardly anybody remembers that exactly the same conclusions were drawn forty years ago on the basis of indirect evidence, and that this evidence was as good forty years ago as it is today. However, it seems to be the general fate of circumstantial evidence that, when more direct evidence turns up (irrespective of whether it is really more "direct" or only apparently so), it is immediately superseded and soon forgotten.
The neuron theory may be now due for certain revisions. The observation of presynaptic dendrites and somata, mainly in Golgi type-2 cells, brings into question the general validity of the principle of "histodynamic polarity"; tight gap junctions, fused membranes with possible loci of specific permeability between different neurons, etc., may call for certain reassessments in the traditional concept. However, it is unlikely that such a revision will invalidate the basic principles of the theory. The antineuronist claims raised in the classical period of the debate have all been shown to be nonrealistic - many even as irrational. If the theory has to be revised, as I am sure it will eventually, the observations for this will come from an entirely different level of investigations and understanding.
1. In publications until 1940 I used my original family name, Schimert.
Identification of Synapses by Experimental Degeneration
Around 1937 my interests shifted to the possibility of using axonal secondary degeneration to trace pathways directly to their synaptic contacts.I was certainly not the first to attempt this, but I had perhaps more luck than most other investigators. Most earlier workers (Hoff, 1932; Hoff and Hoff, 1934; Foerster, Gagel, and Sheehan, 1933) used the Cajal impregnation techniques, but their results were not really convincing (see criticism by Phalen and Davenport, 1937) because the Cajal procedures do not generally stain degeneration fragments of axons; neither did the Bodian and other protein silver stains, although they were otherwise superior to the classical procedures. The only author who could observe unequivocal terminal degeneration was Miskolczy (1931), who looked for the degeneration of the mossy fibers in the cerebellar cortex, which could be well stained in various stages of degeneration with the Cajal methods. In retrospect I do not doubt that Ramón y Cajal would have been the first to exploit this technique in the central nervous system if it were not for the unfortunate circumstance that his silver stain gave unsatisfactory results with degenerating terminal elements.
A successful staining procedure for degenerating terminal axons and synapses actually had been developed much earlier by Rasdolsky (1923, 1925), a neuropathologist from Leningrad, and his pictures and indeed his results were convincing. However, his technique - a modified Alzheimer stain - was extremely cumbersome and involved, and after some unsuccessful attempts (probably due to some difference in the dyes used) I gave the venture up, especially since I was satisfied with the results of the Bielschowsky silver stains (Schimert, 1938b, 1939; Szentágothai-Schimert, 1941). Strangely, when I met Rasdolsky for a few moments many years later in Leningrad, during a memorial meeting at the graveside of I.P. Pavlov on the occasion of the 1949 Pavlov Centennial, he was not even aware of any development in this field. Then an aging man and apparently in failing health, he had some difficulty recalling his own studies when I told him with enthusiasm that his work 25 years earlier had begun to bear fruit. To be exact, my optimism was still not too well founded at that time and was shared by few neuroscientists, because this was long before the eventual "breakthrough" of the "suppressive silver stains" of Nauta and Gygax (1954).
The early history of the axonal and synaptic degeneration method is little known and rarely if ever appropriately cited.2 Nobody could deny that the real breakthrough in the application of experimental axonal degeneration came with the introduction by Nauta and Gygax (1954) of silver stains that make it possible to suppress the staining of normal elements, and their recent modification by Fink and Heimer (1967) (and an array of more recent formulas) for more delicate terminal fibers. However, if criticism is permitted by an old hand at this game, I would have wished that students in this field had not forgotten about the original Bielschowsky stains - especially for identification of the real synaptic terminals - in their enthusiasm over the easy results gained with the modern suppressive stains. The consequence of this neglect was much uncertainty and discussion about whether the fragments corresponded to synaptic terminals or to preterminal fibers. Some of my first drawings, published in 1938 and reproduced here in Figure 6.2, might indicate what I mean.
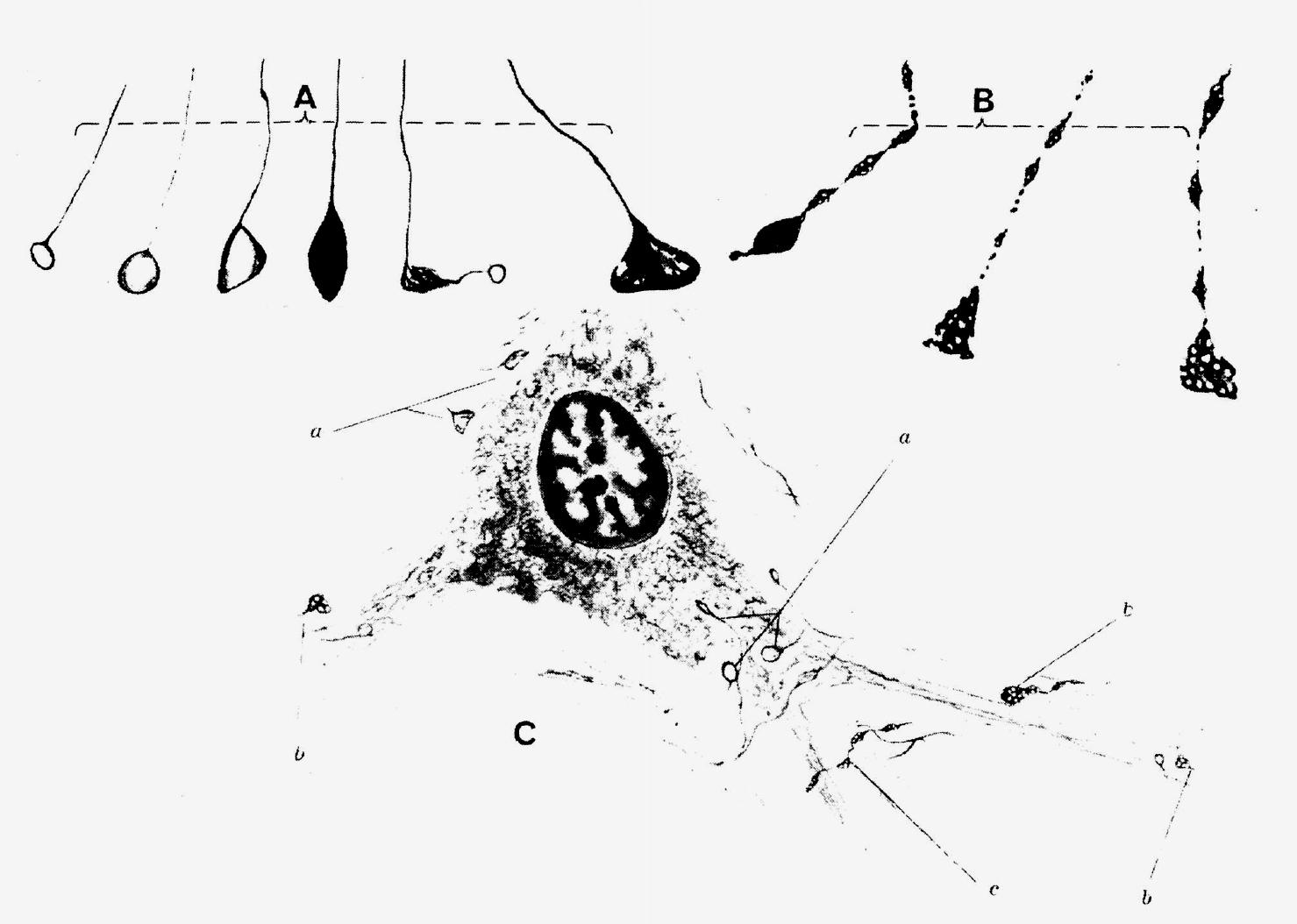
Figure 6.2 Drawings of synaptic degeneration in the spinal cord as revealed by the ordinary
Bielschowsky stain. (A) Normal terminals showing a wide variation of size and staining but a clearly
visible thin preterminal fiber. (B) A degenerated terminal with the characteristic "foamy" appearance
(its equivalent is well recognizable now under the electron microscope), and the clear
fragmentation of the presynaptic axon. (C) The relation to a nerve cell body of normal boutons
(a), degenerated terminals (b), and preterminal fiber (c). From Schimert (1938b).
Although it was difficult to find degenerated terminals among the hundreds of thousands of normals, the few found could be clearly identified as real synaptic contacts.
I did not think it fortunate that, as a result of a modification introduced by Glees (1946), whose work otherwise had considerable merits in the early phase of the degeneration studies, the expression "bouton degeneration technique" became popular for some years before the introduction of the Nauta technique. This expression was based on the appearance of large, heavily stained ringlike structures in the regions of axonal degeneration. We know now from electron microscopy that these are rings of neurofilaments that undoubtedly occur in the so-called filamentous stage of degeneration. But they are not confined to terminals, and they are not characteristic of all neuron types. However, all these minor details have now become irrelevant, since degeneration of synaptic terminals can be better identified under the electron microscope, and degeneration techniques soon will be superseded by the new radioautographic methods using uptake by the cell bodies of tritiated amino acids (Lasek et al., 1968; Cowan et al., 1972).
2. However, I cannot, of course, complain about neglect in the citation of my early papers if a more recent author finds himself in disagreement with one of my minor statements or interpretations.
Stereotaxic Techniques and the Functional Anatomy of Reflex arcs
Luckily, P. von Mihálik had earlier called my attention to the possibilities offered by the stereotaxic technique of Horsley and Clarke (1908), which after a delay of more than 20-25 years had begun to be routinely used by S. W. Ranson and his group in Chicago. Starting in the early forties with my own homemade apparatus (a polar-coordinate apparatus for cats, another for rats built of old microscope stages, and a special instrument for the spinal cord; see Szentágothai, 1951), I began exploring the sites of motoneurons supplying individual muscles. This was not exactly a fortunate choice, as my methods for exploring the somatotopic localization of various muscles by electrical stimulation (Szentágothai, 1942, 1948a, 1949) and also by axonal degeneration after minor lesions had been placed intovarious sites of the nuclei were fraught with many sources of error. I made more blunders in this work than I care to think of, even though Jan Jansen, Sr., tried to caution me in his gentle way - I am afraid with little success - against overrating the capacities of my technique.
However, in spite of the many mistakes and misinterpretations in detail, this work led me to the study of the functional anatomy and physiology of the vestibulo-ocular reflex arc (Szentágothai, 1943, 950a, 1952) and ocular movements (Szentágothai, 1950a). These studies gave me the opportunity to concentrate upon such problems as the functional anatomy of complex reflex arcs and inhibition. This whole story and field is much too involved to be retold here, even in its broadest outlines. Needless to say, I was heavily influenced by the work and ways of reasoning of R. Lorente de Nó (1933), to whom I am greatly indebted for this most stimulating influence. Being able to read the papers by Högyes (1880)3 on the labyrinthine reflexes in the Hungarian original, I also was captured by the fascinating simplicity and beauty in the basic arrangement of this apparatus. As demonstrated by the recent study of Ito (1973), the mechanism is much more complex than could have been imagined in the early fifties, but the basic principles appear still to be valid.
Owing to the low level in the early forties (speaking with some euphemism) of neurophysiology in Hungary, and in fact in most of continental Europe, the textbook of J. F. Fulton (1938) came to me as a revelation. It opened my eyes to the possibility of joining the type of neuroanatomy that I was doing with the results of electrophysiological studies. I cannot now remember why I made stereotaxic lesions in the early forties in the mesencephalic nucleus, but it was not until 1946 that I suddenly realized its significance for the study of the stretch reflex arc. Everything went very smoothly from then on. The first muscle spindle in the masseter muscle I looked at had a clearly degenerated anulospiral terminal, and nice terminal degeneration was found in the mesencephalic nucleus of the trigeminal nerve of the same preparation.
My paper on the anatomical basis of the monosynaptic reflex arc (1948b) was hailed and publicized by J. F. Fulton in the kindest fashion, and I became convinced that this was the course neuroanatomy had to take. Indeed, the search for parallelisms and eventually a dialogue between neuroanatomy and neurophysiology proved to be rewarding, particularly in Clarke's column (Szentágothai and Albert, 1955) and in the spinal motor nucleus (Szentágothai, l958, 1961, 1967b). The latter studies came about under the influence of a fruitful correspondence beginning in 1954 - and later developing into a friendship and collaboration "á distance" - with Sir John Eccles. His book The Neurophysiological Basis of Mind (1953), and subsequent studies on two elementary mechanisms of inhibition (Eccles, Fatt, and Koketsu, 1954; Eccles et a1., 1954), prompted me to try my hand at the synaptic basis of inhibition. Although such attempts at the light-microscopic level were premature, some of their results were essential in preparing the way for the functional anatomical approach to neuron nets. Neurophysiology being far in the lead, I soon incurred the kind criticism that said: "If Sir John makes some claim of a new physiological mechanism, this guy (J.Sz.) soon finds an appropriate anatomical basis. "This was true for the time being, but the time was soon to come when anatomy would take its turn in the forefront. However, first the story of my hypothalamic adventure has to be told.
3. It was a most unfortunate circumstance that Högyes published these studies only in Hungarian and, additionally, in a very heavy and cumbersome style of expression. In the pre-Sherringtonian era it was nearly impossible even for the reader familiar with Hungarian to grasp the fundamental importance of these studies. When the papers were eventually translated into German in 1911, unfortunately without the necessary editorial adaptation to the neurophysiology of the time, they understandably exercised little influence.
Hypothalamic Adventures
Although I had tried to exploit secondary degeneration in the hypothalamus as early as 1943-44, especially in the supra-optico-hypophyseal pathway, which I knew from the classical descriptions and the studies of S. W. Ranson's group at Northwestern University, the results were marginal. I had some notion of the neurosecretory concept of the Scharrers (1937, 1940), and I had the vague idea that it might be rewarding to see how a neurosecretory pathway degenerates. Otherwise my ignorance of hypothalamo-hypophyseal relationships and endocrine mechanisms could not have been more complete. However, when I took over the chair of anatomy at Pécs University in early 1946, I was joined by a young postdoctoral fellow, N.S. Halmi (now at the University of Iowa), who had a remarkable knowledge of the then-emerging literature of this field and a clear idea of what he wanted to do with my stereotaxic instruments. Indeed, the first experiments with hypothalamic (tuberal) lesions produced spectacular changes in the endocrine organs. Although Dr. Halmi left soon afterwards for the United States, where his remarkable studies in this field and in pituitary cytology became well known, some of my young coworkers were already infected with the hypothalamic bug, and, with some reluctance, I had to go along with them.
Due to my ignorance, our progress was very slow and circuitous in the beginning. Foreign travel was out of the question for us in the late forties and early fifties; but gradually my coworkers, Drs. Flerkó, Mess and Halász, developed their own experimental models, some of them quite ingenious and now well known by neuroendocrinologists. Looking back on our early naive exploits, I have the feeling that isolation for some limited time, when a group is left largely to its own intellectual resources, may be quite beneficial. My task on this team was the study of the general neuroanatomy and circulation of the hypothalamo-hypophyseal complex. Since axonal and synaptic degeneration gave very meager results with the methods then available - even with the Nauta stains - I soon had to turn to the Golgi methods.
The Golgi pictures had fascinated me even as a high-school student when, having a student microscope and a hobby-book on microtechniques (but no microtome), it was quite logical to play around with Golgi methods in which the thick sections could be cut with a razor blade. Later, however, as an undergraduate and then research assistant in the anatomy department, I succumbed to the general opinion of the time that nothing new could be expected from Golgi's method after the studies of Ramón y Cajal and other classical authors. The observations on the hypothalamus introduced me to the problems of local connectivity in neuron networks and gave me the feeling that the Golgi method still had much to offer for the understanding of synaptic architecture. This impression was soon strengthened by the remarkable papers on Golgi architecture of the Scheibels (1954, 1955, 1958) and of Fox and Barnard (1957). Still, my observations on the small neurons in the mediobasal hypothalamus, and the identification, in 1962, of the parvicellular tuberoinfundibular neurosecretory system and its terminals in the surface zone of the median eminence, which could be seen so clearly in the Golgi stains, did not impress most neuroendocrinologists (some even thought the whole story ridiculous) until the terminals could be seen under the electron miscroscope. So, eventually, this enterprise turned out quite well, and various editions of the monograph Hypothalamic Control of the Anterior Pituitary, prepared jointly with Drs. Flerkó, Mess, and Halász, were received favorably. Looking back now on this period, I am quite conscious of many naive, romantic features in the book especially in the first edition, for which I have to assume sole responsibility. Drs. Flerkó and Halász were of more realistic disposition and tried to dissuade me from various unorthodox notions. Even so our efforts to explain the results of hypothalamic interferences by various types of hypothalamic feedback (negative, positive, short-circuit, purely neural, etc.) made a modest contribution to the understanding of these complex mechanisms.
The Cerebellar Cortex and the Modular Concept of Neuron Nets
Reverting to the main course of my exploits, I must now tell my side of the cerebellar story. The whole thing started innocently enough with the identification of the climbing fibers as the terminations of the olivocerebellar pathway (Szentágothai and Rajkovits, l959).4 Due to the bad staining qualities of degenerated fibers in the molecular layer of the cerebellum, the evidence was somewhat meagre - as has been pointed out with some relish by critical authors up to this day. However, I still suffer from the misconception that it is the interpretation that counts and not whether the finding is obvious and beautifully demonstrated, or is barely recognizable. I have told the long and romantic story of the climbing fibers elsewhere (Eccles, Ito, and Szentágothai, 1967: pp. 37-38), so I need not repeat it here. This finding gave considerable leverage to the application of microelectrophysiological methods to the cerebellar cortex by Sir John Eccles and his associates.
My present approach to the functional anatomy of neuron nets began when I was trying to convey to my undergraduate medical students, in the usual course on neuroanatomy, an idea about how excitation may spread in a piece of cerebellar cortex. Since specific inhibitory interneurons were not yet known, I speculated at the blackboard5 that excitation might spread along the longitudinal axis of the folium via the parallel fibers, thought then to be about 3 mm in length, and across the folium via the basket axons (i.e., about 1 mm to both sides of a simultaneously excited beam of parallel fibers). When the concept of specific inhibitory interneurons gradually became established and generalized in the minds of neuroscientists in the late fifties, I suddenly realized that this speculation on the cerebellar cortex would become more elegant if the basket cells were endowed with inhibitory properties.
The Cerebellar Cortex and the Modular Concept of Neuron Nets
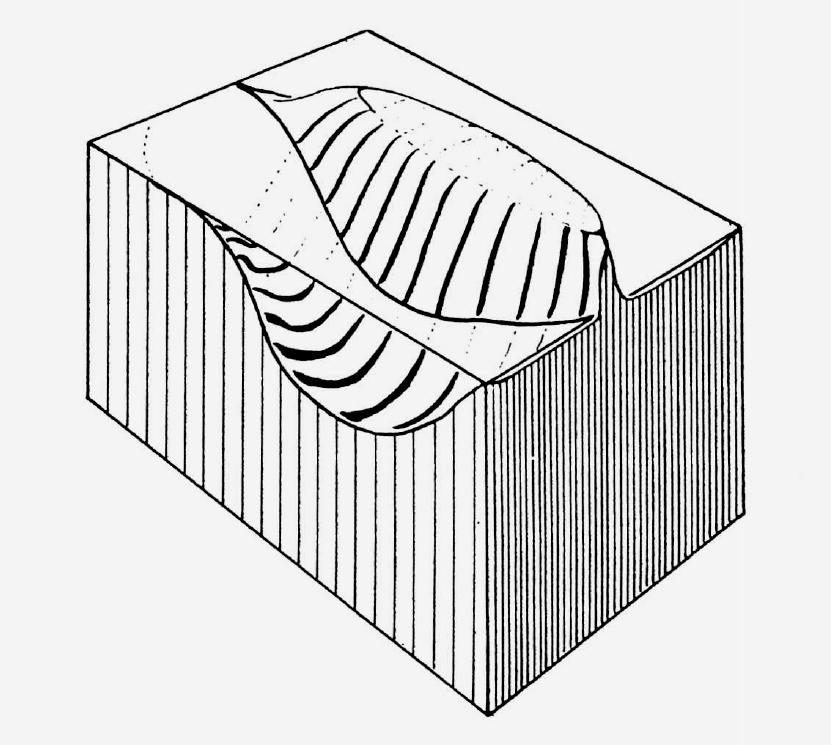
Figure 6.3 Pictorial representation of the effect on Purkinje-cell activity of a bundle of fibers
imagined to run parallel to the longitudinal axis of the brick-shaped piece of molecular layer
illustrated. The field of excited Purkinje cells is represented as a "mountain ridge" standing out
from the plane, which symbolizes the resting state. In the foreground, the depressed state of activity -
due to the action of the basket cells - is represented as a depression of the ground running
parallel to the ridge.
Reverting to the main course of my exploits, I must now tell my side of the cerebellar story. The whole thing started innocently enough with the identification of the climbing fibers as the terminations of the olivocerebellar pathway (Szentágothai and Rajkovits, l959).4 Due to the bad staining qualities of degenerated fibers in the molecular layer of the cerebellum, the evidence was somewhat meagre - as has been pointed out with some relish by critical authors up to this day. However, I still suffer from the misconception that it is the interpretation that counts and not whether the finding is obvious and beautifully demonstrated, or is barely recognizable. I have told the long and romantic story of the climbing fibers elsewhere (Eccles, Ito, and Szentágothai, 1967: pp. 37-38), so I need not repeat it here. This finding gave considerable leverage to the application of microelectrophysiological methods to the cerebellar cortex by Sir John Eccles and his associates.
My present approach to the functional anatomy of neuron nets began when I was trying to convey to my undergraduate medical students, in the usual course on neuroanatomy, an idea about how excitation may spread in a piece of cerebellar cortex. Since specific inhibitory interneurons were not yet known, I speculated at the blackboard5 that excitation might spread along the longitudinal axis of the folium via the parallel fibers, thought then to be about 3 mm in length, and across the folium via the basket axons (i.e., about 1 mm to both sides of a simultaneously excited beam of parallel fibers). When the concept of specific inhibitory interneurons gradually became established and generalized in the minds of neuroscientists in the late fifties, I suddenly realized that this speculation on the cerebellar cortex would become more elegant if the basket cells were endowed with inhibitory properties.
(Perhaps my readers will not be unduly shocked if I confess that the elegance and beauty of a concept is much more attractive to me than the dreary details of fact.)6 Eventually I gave a formal lecture on this at our Academy at the beginning of 1963. In Figure 6.3 the pictorial representation of the concept (published only in the Hungarian report of this lecture) is reproduced. The figure gives an overall view of a field of excited Purkinje cells in the form of an elongated mountain (corresponding to the longitudinal course of the parallel fibers), with the valleys on either side representing the regions where the Purkinje cells are inhibited by the basket cells. I will not give the details of the concept because they have been reproduced on several occasions and publicized by Sir John (see Szentágothai, 1963: Figure 4; 1965: Figure 19; Eccles, Ito, and Szentágothai, 1967: Chapter 12, Figure 115).
The rationale of this approach was that, knowing the anatomy of any neuron network, one might make arbitrary assumptions about the excitatory or inhibitory nature of the various neuron types constituting the network and then try to understand how such a network might function under the assumed circumstances. This concept of a "neuronal machine" in the cerebellar cortex worked very nicely as long as local stimulation was applied to the cerebellar cortex, probably because local stimulation was essentially a repetition of the original anatomical speculation. Difficulties were soon encountered when researchers in this field began to apply more physiological conditions, and the recent studies of Eccles and coworkers (1971) and Llinás, Precht, and Clarke (1971) have shown clearly that closely neighboring Purkinje cells may behave in very different ways in the same situation, something not foreseen in the original, simplified concept.
My own peace of mind (or "tao" in the sense of Lao-Tse) in this matter had been disturbed before by the uneasy feeling that any speculation on the possible functions of neural nets would be on extremely shaky ground unless exact numerical and metrical relations of the neuron network were known. We started, therefore, a major enterprise in stereological analysis of the cerebellar cortex. The results (Palkovits, Magyar, and Szentágothai, 1971a,b,c, 1972) were shocking at first, because they showed that all numerical and many metrical conclusions obtained from earlier naive counts and measurements were grossly off the mark. On second thought, however, the accumulated data, and especially the total numerical transfer functions of the cerebellar neuron chain, make some sense and offer distinct possibilities for realistic modeling of the network functions by digital computer. For this purpose, each of the sequential links of the neuron chain have only to be compressed into a two-dimensional matrix (Szentágothai and Arbib, 1974: Figure 19) with the correct positions and the relative densities of the several elements. Any arbitrary input can then be investigated by the computer as it is successively processed through the stacked two-dimensional matrices (Pellionisz and Szentágothai, 1973,1974). This modeling leads to most interesting predictions that I cannot go into here. It might suffice to mention that closely neighboring Purkinje cells behave in very different ways to the same input, exactly as found by the physiologists.
This example does not by any means show that the type of reasoning about neural networks that led originally to the neuronal-machine concept is useless or basically false. On the contrary, it is the only way to look at neuron networks from the viewpoint of functional anatomy. One simply has to be more cautious in drawing far-reaching functional conclusions from the apparent overall features of the network. For example, the apparent features of the neuron network of the cerebellar cortex are a longitudinal spread of excitation and a transverse spread of inhibition. But if all the conditions are considered - something only the computer can do because of the large number of elements and variables - it emerges that any focal excitation reaching the granular layer by the mossy fibers can be conveyed to, and brought into interaction with, neighboring foci in the longitudinal and the transversal axes of the folium as well.
My anatomical speculations did not remain confined to the cerebellar cortex. I tried to generalize this way of looking at neuron nets into a general concept of "higher integrative units of the nervous system" (Szentágothai, 1967a). By this term I meant the minimum amount of neural tissue that would be able to perform the essential part of the information processing for which that piece of tissue is built. Now, after six years, these attempts look shockingly naive to me and might appear even more so to others. I cannot say more in their defense than that, however crude these models might be, they still offered stepping-stones over which some progress could be made. Consider, for example, the "iterative process" by which this basic circuit model of the neocortex could gradually be approached (Szentágothai, 1969, 1970, 1971, 1972a,b, 1973a,b). I do not for a moment believe that even the most recent of my circuit models (still unpublished) can make any claim to be "correct," but it is a way to raise questions and focus assumptions that can be rested by neurohistology and eventually by physiology.
By a fortunate convergence, the important concept, first raised by the Scheibels (1958), of a quasi-"modular" structure of the nervous tissue will soon be joining this general line of neuroanatomical reasoning. Considering the spinal segmental apparatus as a sequence of transversely oriented modules of neuropil (Scheibel and Scheibel, 1968; Szentágothai and Réthelyi, 1973) - something like stacked "chips" or microintegrated circuits in modern electronics technology - might prove eventually to be an important structural framework for the better understanding of nervous system design (see Szentágothai and Arbib, I974). Similar modules of structure can be recognized everywhere in the neural centers. The cerebellar cortex itself is the best example of a modular design on the cellular level (Szentágothai and Arbib, 1974: Figure 42A). Here the separation of the arborisation spaces of the Purkinje cells automatically brings about a regularity of space arrangement that results in a whole chain of regularities and in the final "quasicrystalline" structure of the network. Similar regularities can be detected in seemingly irregular entanglements of dendritic and axonal arborizations, where the repetition at regular intervals of arborizations that are in themselves irregular leads to interference (or moiré) patterns, as I have tried to show for the lateral geniculate body (Szentágothai, 1972b, 1973b). There is a continuous line of these structures, from elementary modules up to large organoid modules of neural structure such as the cortical barrels of Woolsey and Van der Loos (1970). We may still be at the very beginning of the path to the understanding of neural nets, but I am convinced that this will be one of the most fruitful routes neuroanatomy can take.
It would be impossible, for obvious reasons, to tell here the detailed story of the developments in synaptology that went parallel with the quest for the understanding of neuronal nets. In the last fifteen years these developments were intimately tied up with the discovery of ultrastructural architectonics in synaptic systems through the use of the electron microscope. My own attitude and role here were determined by my fascination with the essence and the possible functional significance of the design and by my lack of interest in, and patience for, detail. I do not want to find excuses for the many mistakes, misinterpretations, or premature conclusions that I made and might have avoided with a more restrained attitude. Nor do I think that excuses are necessary, because a more imaginative approach - even though burdened with a broad margin of error - sometimes does more for progress than the "correct" inductive path to discovery. To be exact, both are needed. I might perhaps return to the example of the glomerular synapses in the lateral geniculate body, which I predicted purely on the basis of light-microscopic studies in a lecture at Atlantic City in April 1961 (Figure 6.4; the original diagram was in color so this figure does not do full justice to the original).
Outlook
My basic stand, for the time being, is a firm conviction that the real breakthrough that will bridge the still widening gap between the "behavioural" (whatever this includes, from simple conditioned reflexes, through memory and learning, to complex built-in behavior patterns and psychology and even up to the phenomenon of consciousness) and the "cellular" (including anatomy, physiology, biochemistry, molecular neurobiology, etc.) hinges on an understanding of the neuron networks. Whatever the molecular mechanisms of the elementary memory trace (most probably something in the material-producing machinery of the neuron), and whatever still unknown molecular or even charge-transfer or cooperative trickery lies behind the mechanism of "plasticity" in synaptic transmission (transduction, etc.), I believe that nature would not have brought forth the neuron network if the basic riddle and/or solution of behavior lay somewhere else. The immense degree and complexity of internal connectivity in the higher integrative centers, hypothalamus, and the limbic structures, striatum, and especially the cerebral cortex, gives me the strong feeling that single (or groups of) neurons may drag into action neighboring ones (or groups) not immediately connected with the chain of neurons to which the input is delivered. This concept can be imagined pictorially in very crude analogy to "flow patterns'" of fluids (Bénard patterns or Zhabotinsky patterns; see Katchalsky, Rowland, and Blumenthal, 1974). Here I must give my tribute to the late Aharon Katchalsky who, in the last scientific meeting that he attended, raised this issue as a case analogous to cooperative phenomena. In this instance, again, we are treading in almost complete darkness, but, nevertheless, some of us may have the feeling that it is here that the solution of the ultimate riddle, consciousness, will be found. The tasks we are facing are well beyond the means and understanding as well as the technical apparatus of the biologist. What we need is the cooperation of theoreticians of various branches. Therefore, I am full of gratitude to the man - in honor of whom this symposium has been called together - who has founded in the Neurosciences Research Program a place where, and an apparatus with whose help, the students of these various fields can meet.
Trying to evaluate, as a function of my own personality, my own humble role in the development of the neurosciences in the last few decades, I am made painfully aware of my inadequacies. However, I have enough sense of humor and resignation to visualize myself as a romantic whose life - in spite of its outward smoothness and largely undeserved success - has been an uninterrupted sequence of quixotic ideas and enterprises, full of premature attempts and mistakes ? some of which might perhaps be excused by the limitations in my circumstances (in equipment and technical resources) - more appropriate sometimes for one of the classic films of Charlie Chaplin than for the biography of a scientist. Or, on a more metaphorical level, I have to accept for my own fate the words of Saint Paul in the Epistle to the Romans (9:16): "It is not to those who will, neither to those who run, but to the mercy of God."
References
Boeke, J. (1926): Die Beziehungen der Nervenfasern zu den Bindegewebselementen und Tastzellen. Das periterminale Netzwerk der motorischen und sesibeln Nervenendigungen, seine morphologische und physiologische Bedeutung, Entwicklung und Regeneration. (Z. Mikrosk. Anat. Forsch. 4:448-509.
Boeke, J. (1933a): Innervationsstudien. III. Die Nervenversorgung des M. ciliaris und des M. sphincter iridis bei Säugern und Vögeln. Ein Beispiel plexiformer Innervation der Muskelfasern. (Z. Mikrosk. Anat. Forsch. 33 :233-275.
Boeke, J. (1933b): Innervationsstudien. IV. Die efferente Gefässinnervation und der sympathische Plexus im Bindegewebe. (Z. Mikrosk. Anat. Forsch. 33:276-328.
Boeke, J. (1939): Innervationsstudien. X. Sympathischer Grundplexus und Bindegewebsstrukturen (Reticulinfasern des Bindegewebes und des Sarkolemmas). (Z. Mikrosk. Anat. Forsch. 46:488- 519.
Cowan, W. M., Gottlieb, D. I., Hendrickson, A. E., Price,J. L., and Woolsey, T. A. (1972): The autoradiographic demonstration of axonal connections in the central nervous system. Brain Res. 37:21-51.
Eccles, J. C. (1953): The Neurophysiologicat Basis of Mind. The Principles of Neurophysiology. Oxford: Clarendon Press.
Eccles, J. C., Faber, D. S., Murphy, J. T., Sabah, N. H., and Táboriková, H. (1971): Investigations on integration of mossy fiber inputs to Purkinje cells in the anterior lobe. Exp. Brain Res. 13:54-77.
Eccles, J. C., Fatt, P., and Koketsu, K. (1954): Cholinergic and inhibitory synapses in a pathway from motor-axon collaterals to motoneurones. J. Physiol. 126:.524-562.
Eccles, J. C., Fatt, P., Landgren, S., and Winsbury, G.J. (1954): Spinal cord potentials generated by volleys in the large muscle afferents. J. Physiol. 125:590-606.
Eccles, J. C., Ito, M., and Szentágothai, J. (1967): The Cerebellum as a Neuronal Machine. New York : Springer-Verlag.
Fink, R. P., and Heimer, L. (1967) : Two methods for selective silver impregnation of degenerating axons and their synaptic endings in the central nervous system. Brain Res.4:369-374.
Foerster, O., Gagel, O., and Sheehan, D. (1933): Veränderungen an den Endösen im Rückenmark des Affen nach Hinterwurzeldurchschneidung. (Z. Anat. Entwicklungsgesch. 101:553-565.
Fox, C. A., and Barnard, J. W. (1957): A quantitative study of the Purkinje cell dendritic branchlets and their relationship to afferent fibres. J. Anat. 91:299-313.
Fulton, J.F. (1938) Physiology of the Nervous System. NewYork:OxfordUniversityPress.
Glees, P. (1946): Terminal degeneration within the central nervous system as studied by a new silver method. J. Neuropathol. Exp. Neurol. 5:54-59.
Hoff, E. C. (1932): Central nerve terminals in the mammalian spinal cord and their examination by experimental degeneration. Proc, R. Soc. B. 111:175-188.
Hoff, E. C., and Hoff H. E. (1934): Spinal terminations of the projection fibres from the motor cortex of primates. Brain 57:454-474.
Högyes, A. (1880): Az akaratlan együttjáró (associált) szemmozgások idegmechanizmusáról [On the nervous mechanism of the involuntary associated movement of the eyes]. Orv. Hetil. 23:17-29.
Horsley, V., and Clarke, R. H. (1908) : The structure and functions of the cerebellum examined by a new method. Brain 31:45-124.
Ito, M. (1973): The vestibulo-cerebellar relationships: Vestibulo-ocular reflex arc and flocculus. In: Handbook of Sensor Physiology Vol. 6. Vestibular System. Kornhuber, H.H., ed. New York: Springer-Verlag.
Katchalsky, A. K., Rowland, V., and Blumenthal, R. (1974): Dynamic patterns of brain cell assemblies. Neurosci. Res. Program Bull. 12:1-187.
Lasek, R., Joseph, B. S., and Whitlock, D. G. (1968): Evaluation of a radioautographic neuroanatomical tracing method. Brain Res. 8:319-336.
Lawrentjew, B.J. (1934): Experimentell-morphologische Studien über den feineren Bau desautonomen Nervensystems. IV. Weitere Untersuchungen über die Degeneration und Regeneration der Synapsen. (Z. Mikrosk. Anat. Forsch. 35:71-118.
Llinás, R., Precht, W., and Clarke, M. (1971): Cerebellar Purkinje cell responses to physiological stimulation of the vestibular system in the frog. Exp. Brain Res. 13:408-431.
Lorente de Nó, R. (1933): Vestibulo-ocular reflex arc. Arch. Neurol. Psychiatr. 30:245-291.
Miskolczy, D. (1931): Über die Endigungsweise der spinocerebellaren Bahnen. (Z. Anat. Entwicklungsgesch. 96 :537 -542.
Nauta, W.J. H., and Gygax, P. A. (1954): Silver impregnation of degenerating axons in the central nervous system: A modified technique. Stain Technol. 29:91-93.
Palkovits, M., Magyar, P., and Szentágothai, J. (1971a): Quantitative histological analysis of the cerebellar cortex in the cat. I. Number and arrangement in space of the Purkinje cells. Brain Res. 32:1-13.
Palkovits, M., Magyar, P., and Szentágothai, J. (1971b): Quantitative histological analysis of the cerebellar cortex in the cat. II. Cell numbers and densities in the granular layer. Brain Res.32:15-30.
Palkovits, M., Magyar, P., and Szenuígothai, J. (1971c): Quantitative histological analysis of the cerebellar cortex in the cat. III. Structural organization of the molecular layer. Brain Res.34:1-18.
Palkovits, M., Magyar, P., and Szentágothai, J. (1972): Quantitative histological analysis of the cerebellar cortex in the cat. IV. Mossy fiber-Purkinje cell numerical transfer. Brain Res. 45: 15-29.
Pellionisz, A., and Szentágothai, J. (1973): Dynamic single unit simulation of a realistic cerebellar network model. Brain Res. 49:83-99.
Pellionisz, A., and Szentágothai, J. (1974): Dynamic single unit simulation of a realistic cerebellar network model. II. Purkinje cell activity within the basic circuit and modified by inhibitory systems. Brain Res. 68: 19-40.
Phalen, G. S., and Davenport, H. A. (1937): Pericellular endbulbs in the central nervous system of vertebrates. J. Comp. Neurol. 68:67-81.
Rasdolsky, J. (1923): Über die Endigung der extraspinalen Bewegungssysteme im Ruckenmark. (Z. Gesamte Neurol. Psychiatr. 86:361-374.
Rasdolsky, J. (1925): Beiträge zur Architektur der grauen Substanz des Rückenmarks (Unter Benutzung einer neuen Methode der Färbung der Nervenfasernkollateralen). Virchows Arch. (Pathol. Anat.) 257:356-363.
Scharrer, E., and Scharrer, B. (1937): Über Drüsen-Nervenzellen und neurosekretorische organe bei Wirbellosen und Wirbeltieren. Biol. Rev.12:185-216.
Scharrer, E., and Scharrer, B. (1940): Secretory cells within the hypothalamus. Res. Publ. Assoc. Res. Nerv. Ment. Dis. 20:170-194.
Scheibel, M. E., and Scheibel, A. B. (1954): Observations on the intracortical relations of the climbing fibers of the cerebellum. A Golgi study. J. Comp. Neurol. 101:733-763.
Scheibel, M. E., and Scheibel, A. B. (1955): The inferior olive. A Golgi study. J. Comp. Neurol. 102:77-131.
Scheibel, M. E., and Scheibel, A. B. (1958): Structural substrates for integrative patterns in the brain stem reticular core. In: Reticular Formation of the Brain. Jasper, H. H., Proctor, L. D., Knighton, R. S., Noshay, W. C., and Costello, R. T., eds. Boston: Little, Brown, pp. 31-55.
Scheibel, M. E., and Scheibel, A. B. (1968): Terminal axonal patterns in cat spinal cord. II. The dorsal horn. Brain Res.9:32-58.
Schimert, J. (1936a): Untersuchrrngen über den Ursprung und die Endausbreitung der Nerven der Iris. (Z. Zellforsch. Mikrosk. Anat. 25:247-258.
Schimert,J. (1936b): Der Nervus intermedius und das Ganglion geniculi nervi facialis. (Z. Mikrosk. Anat. Forsch. 39:35-44.
Schimert, J. (1937): Die Nervenversorgung des Myokards. (Z. Zeffirsch. Mikrosk. Anat. 27:246-266.
Schimert, J. (1937-1938): Discussion of paper by J. Boeke, Dritte Wissenschaftliche Sitzung. Verh. Anat. Ges. 45-46: 158-159.
Schimert, J. ( 1938a): Die Endigungsweise des Tractus vestibulospinalis. (Z. Anat. Entwicklungsgesch. 108:761-767.
Schimert, J. (1938b): Die "Syncytielle Natur" des vegetativen Nervensystems. Z. Mikrosk. Anat. Forsch. 44:85-119.
Schimert, J. (1939): Das Verhalten der Hinterwurzelkollateralen im Rückenmark. (Z. Anat. Entwicklungsgesch. 109 :665-687.
Stöhr, P., Jr. (1935): Beobachtungen und Bemerkungen über die Endausbreitung des vegetativen Nervensystems . (Z. Anat. Entwicklungsgesch. 104: 133- 158.
Stöhr, P., Jr. (1939): Über "Nebenzellen" und deren Innervation in Ganglien des vegetativen Nervensystems, zugleich ein Beitrag zur Synapsenfrage. Z. Zellforsch. Mikrosk. Anat. 26:569-612.
Szentágothai, J. (1942): Die innere Gliederung des Oculomotoriuskernes. Arch. Psychiatr. 115:127-135.
Szentágothai, J. (1943): Die zentrale Innervation der Augenbewegungen. Arch. Psychiatr. 116:721-760.
Szentágothai, J. (1948a): The representation of facial and scalp muscles in the facial nucleus. J. Comp. Neurol. 88: 207-220.
Szentágothai, J. (l948b): Anatomical considerations of monosynaptic reflex arcs. J. Neurophysiol. 11: 445-454.
Szentágothai, J. (1949): Functional representation in the motor trigeminal nucleus. J. Comp. Neurol. 90:111-120.
Szentágothai, J. (1950a): The elementary vestibulo-ocular reflex arc. J. Neurophysiol. 13:395-407.
Szentágothai, J. (1950b): Recherches expérimentales sur les vois oculogyres. Sem. Hop. Paris 26: 2989-2995.
Szentágothai, J. (195l1): Short propriospinal neurons and intrinsic connections of the spinal gray matter. Acta Morphol. Acad. Sci. Hung. 1:81-94.
Szentágothai, J. (1952): Die Rolle der einzelnen Labyrinthrezeptoren bei der Orientation zum Augen und Kopf im Raume. Budapest: Akadémiai Kiadó.
Szentágothai, J. (1958): The anatomical basis of synaptic transmission of excitation and inhibition in motoneurons. Acta Morphol. Acad. Sci. Hung. 8:287-309.
Szentágothai, J. (1961): Anatomical aspects of inhibitory Pathways and synapses. In: Nervous Inhibition (Proceedings of the Second Friday Harbor Symposium). Florey, E., ed. Oxford: Pergamon Press, pp.32-45.
Szentágothai, J. (1963): Ujabb adatok a synapsis funkcionális anatómiájához [New data on the functional anatomy of synapses] . Magy. Tudom. Akad. Biol. Orv. Tudom. Osztal. Közl. 6: 217-227.
Szentágothai,J. (1965): The use of degeneration methods in the investigation of short neuronal connexions. Prog. Brain Res. 14:1-32.
Szentágothai, J. (1967a): The anatomy of complex integrative units in the nervous system. In: Recent Developments of Neurobiologl in Hungary. Vol. I. Results in Neuroanatomy, Neurochemistry, Neuropharmacology and Neurophysiolog. Lissák, K., ed. Budapest: Akadémiai Kiadó, pp. 9-45.
Szentágothai, J. (1967b): Synaptic architecture of the spinal motoneuron pool. Electroencephalogr. Clin. Neurophysiol. Suppl. 25:4-19.
Szentágotlrai, J. (1969): Architecture of the cerebral cortex. In: Basic Mechanisms of the Epilepsies. Jasper, H. H., Ward, A. A., Jr., and Pope, A., eds. Boston: Little, Brown, pp. 13-28.
Szentágothai, J. (1970): Les circuits neuronaux de l'écorce cérébrale. Bull. Acad. R. Med. Belg. 10:475-492.
Szentágothai, J. (1971): Some geometrical aspects of the neocortical neuropil. Acta Bíol. Acad. Sci. Hung. 22:107-124.
Szentagothai, J. (1972a) : The basic neuronal circuit of the neocortex. In: Synchronization of EEG Activity in Epilepsies (Symposium Organized by the Austrian Academy of Sciences, Vienna, September 12-13, 1971). Petsche, H., and Brazier, M. A. B., eds. Vienna: Springer-Verlag, pp. 9-24.
Szentágothai, J. (1972b): Lateral geniculate body structure and eye movement. Bibl. Ophthalmol. 82: 178-188.
Szentágothai, J. (1973a): Neuronal and synaptic architecture of the lateral geniculate nucleus. In: Handbook of Sensory Physiology. Vol. VII/3B. Central Processing of Visual Information. Jung, R., ed. Berlin: Springer-Verlag, pp. 141-176.
Szentágothai, J. (1973b): Synaptology of the visual cortex. In: Handbook of Sensory Physiology. Vol. VII/3B. Central Processing of Visual Information. Jung, R., ed. Berlin: Springer-Verlag, pp. 269-324.
Szentiagothai-Schimert, J. (1941): Die Endigungsweise der absteigenden Rückenmarlrsbahnen. (Z. Anat. Entwicklungsgesch. 111: 322-330.
Szentágothai, J., and Albert, Á. (1955): The synaptology of Clarke's column. Acta Morphol. Acad. Sci. Hung. 5:43-51.
Szentágothai, J., and Arbib, M. A. (1974): Conceptual models of neural organization. Neurosci. Res. Program Bull. 12:305-510.
Szentágothai, J., Flerkó, B., Mess, B., and Halász, B. (1962) : Hypothalamic Control of the Anterior Pituitary. An Experimental-Morphological Study. Budapest: Akadémiai Kiadó (2nd ed., 1965; 3rd ed., 1968).
Szentágothai, J., and Rajkovits, K. (1959): Übe. den Ursprung der Kletterfasern des Kleinhirns. (Z. Anat. Entwicklungsgesch. 121: 130-141.
Szentágothai, J., and Réthelyi, M. (1973): Cyto- and neuropil architecture of the spinal cord. In: Developments in Electromyography and Clinical Neurophysiology. Vol. 3. Desmedt, J. E., ed. Basel: S. Karger, pp. 20-37.
Woolsey, T.A., and Van der Loos, H. (1970): The structural organization of layer IV in the somatosensory region (SI) of mouse cerebral cortex. The description of a cortical field composed of discrete cytoarchitectonic units. Brain Res. 17:205-242.